C P Snow’s lecture ‘The Two Cultures’ (1959) argued that the perceived divide between scientists and literary scholars is narrower than commonly believed. They both fundamentally seek to understand and express the relationships that structure reality – whether human relationships in literature, or physical relationships in science.
In 1961, on the heels of that lecture, a children’s book came out – The Phantom Tollbooth by Norton Juster, a funny, punny allegorical fantasy that made the same argument but in a way that captivated readers well into the 1990s, when I first encountered this story: Milo, a boy already besieged by adult-like ennui and existential despair, takes on the quest to bring back the princesses Rhyme and Reason, reuniting them with their two quarrelsome brothers: King Azaz the Unabridged, Ruler of Dictionopolis, and the Mathemagician, Ruler of Digitopolis.
King Azaz claims that words are superior to numbers; the Mathemagician insists the reverse. In the end, the brothers reconcile and rebuild the City of Wisdom with the help of Rhyme and Reason, and Milo returns to his own world with renewed curiosity for words and numbers. By age 13, I’d already been convinced of the value of interdisciplinarity.
Eventually, I would learn that stories are not just a way of communicating science; they are intrinsic to science, actually part of doing science. My own story of merging these Two Cultures – for me, literary writing and particle physics – was complicated by a Third Culture, religion. I grew up in Utah, in an era when Mormon women could have physics careers, technically, but following this path was difficult, lonely, and considered a threat to the traditional family model. We were encouraged to pursue education, not to prepare for competitive careers but for traditional roles as wives and mothers. This worldview, where a woman’s education is merely a safeguard if her husband can’t work, exemplifies what George W Bush’s speechwriter Michael Gerson called ‘the soft bigotry of low expectations’. It is a mindset that stifles ambition and curiosity. In fact, in my world, ambition in a woman signified pride, selfishness, sin.
Yet I loved my advanced high-school mathematics and physics classes. With friends, I won team competitions in physics and computer programming. As a teenager, I even interned for three summers with the Cosmic Ray Research Group at the University of Utah – the High Resolution Fly’s Eye collaboration that detected the Oh-My-God particle in 1991. This rare ultrahigh-energy cosmic ray – probably a proton – was an atomic shard travelling close to the speed of light, bombarding our detector with an absurd amount of energy. This event defied physics models and opened new questions about the limits of energy in the Universe, presenting a cosmic mystery story I wanted to pursue.
Despite my interest in cosmic rays, the Third Culture reigned supreme. The pressure to conform was invisible but visceral: during my first semester at Utah’s Brigham Young University (BYU) in 2002, led not by reason or rhyme but by a fear of angering God and my Church, I walked out of the introductory physics class – the only woman in attendance – and changed my major from astrophysics to English. Burying myself in stories and syntax, I felt sad about the physics but decided to make the most of my education before I married. BYU’s editing and linguistics courses were truly superb, and I learned to find patterns in natural language, and improve those patterns to benefit readers and increase the quality of communication. Editing, I thought, was something I could do from home with a family. Maybe I’d even dare to be a science editor.
Fast-forward 10 years, and that’s exactly what I was doing, while my toddler slept. I loved reading upper-level STEM textbooks as a freelance editor for Taylor & Francis; it was as physics-adjacent as I could manage. I could search the pattern of writing for errors while absorbing the patterns of mathematics and physics, even if I didn’t understand it perfectly.
But I wanted to, though the desire still felt dangerous. I started writing fiction and essays, and my frustrations seethed onto the page. As soon as my son woke up, however, I would focus on him. Like me, he had a natural affinity for both letters and numbers, and we spent hours laughing and learning together. His intense curiosity reignited my own.
In October 2012, still wrestling with deeply ingrained but self-limiting patterns of thought, I interviewed the psychologist LaNae Valentine, who directed the BYU Women’s Services and Resources Center. She told me that the counsellors for college women were explicitly instructed to use the word ‘education’ instead of ‘career’ – an omission reflected in the name of the centre itself. It grated on her, she said, but she complied.
In the midst of disaster, I came full circle, back to the beginning of my story
The explicit omission was a revelation to me. Second-wave feminism had come and gone, but its reverberations were reaching me for the first time. My husband read Simone de Beauvoir’s The Second Sex (1949), liked reading it, and handed it to me, which started a tsunami of good, hard questions. What was I good at, drawn to, excited by? Was it too late to develop previously abandoned skills? Confronting the self-limiting story from my Third Culture led to a breakthrough: like Andrew, I could take myself and my career seriously, and still be a great spouse and parent.
Over the next 10 years, I began to level up in writing, then science writing, then physics. I contended with a Fourth Culture: life as the spouse of a US Foreign Service Officer. Moving from Washington, DC to the Marshall Islands, then Montreal, Virginia and Nicaragua, I had to actively resist the feelings of loss that come for those supporting a spouse’s job abroad. Fortunately for me, Andrew supported my personal and professional ambitions in return, so I could thrive alongside his career even as we moved country every two or three years. I started publishing science essays and teaching science writing at Johns Hopkins University in Maryland, both of which could be done remotely.
In April 2018, political violence erupted in Nicaragua, and embassy families were sent back to safety in the US. Max and I evacuated to Utah while Andrew remained in Managua as essential personnel. Making the sweetest lemonade with the bitterest of lemons, I returned to work for the Cosmic Ray Research Group, now known as the Telescope Array Project. In the midst of disaster, I came full circle, back to the beginning of my story.
From left: John Matthews of the Telescope Array, the author Jamie Zvirzdin and her former supervisor, Stan Thomas, at a café at the University of Utah
I have been making up for lost time ever since. I couldn’t influence a dictator in Nicaragua, but I could traipse out to the Utah desert, fix detectors, and operate telescopes to help solve the mystery of ultrahigh-energy cosmic rays. Reunited with Andrew in October 2018 following his time in Nicaragua, I picked up my work for the Telescope Array Project remotely from Maryland, writing programs, analysing data and even operating telescopes during night shifts from my work computer. I am now more than halfway through a Master’s in applied physics from Johns Hopkins, a remote programme I can pursue from our current post in Germany.
My unconventional path to physics reveals an important insight for those who may feel excluded from the field or intimidated by its complexities: at its core, physics is fundamentally a word problem. A story problem. Personal stories, history stories, thought experiments, formal proofs, metaphors, cautionary tales: surround yourself with the various stories embedded in physics, and you’ll find firm footing wherever you tread in this field.
Some of the best physicists and physics teachers are also great storytellers: they tell wild tales of things that happened to them, both real and perhaps slightly embellished for comedic effect.
One such tale comes from my friend Jihee Kim, now a postdoc at Brookhaven National Laboratory in New York. As a new PhD student with the Telescope Array collaboration, she was asked to take a picture of one of our fluorescence detectors. Housed in dark sheds, these detectors use large mirrors to capture faint ultraviolet light produced by cosmic-ray showers in the atmosphere during moonless nights. Not realising the potential danger, Kim opened the shed doors a little to let in more afternoon light for the photo. Almost immediately, she smelled something burning – indirect light from the Sun had reflected off the mirror and was now focused, like a magnifying glass, onto a nearby cable.
To make sure no one else made the same mistake, our boss, John Matthews, put up DANGER signs in black, red and white, warning students never to let sunlight touch the telescope mirrors. He added a picture of the melting face from the film Raiders of the Lost Ark – just in case anyone needed an extra reminder.
The history-based stories we tell in physics model the scientific method itself
We need to hear stories of people who surmount difficulties large and small, who push past ennui and cynicism and embarrassment and discouragement, who act with honesty and courage, who humbly ask for and receive help, to advance the frontline of knowledge. I hope my story will spur more women and minorities to take the best of the cultures they belong to and give themselves permission to enter academic gates they thought were closed to them. Work hard and work smart, and record your stories for others.
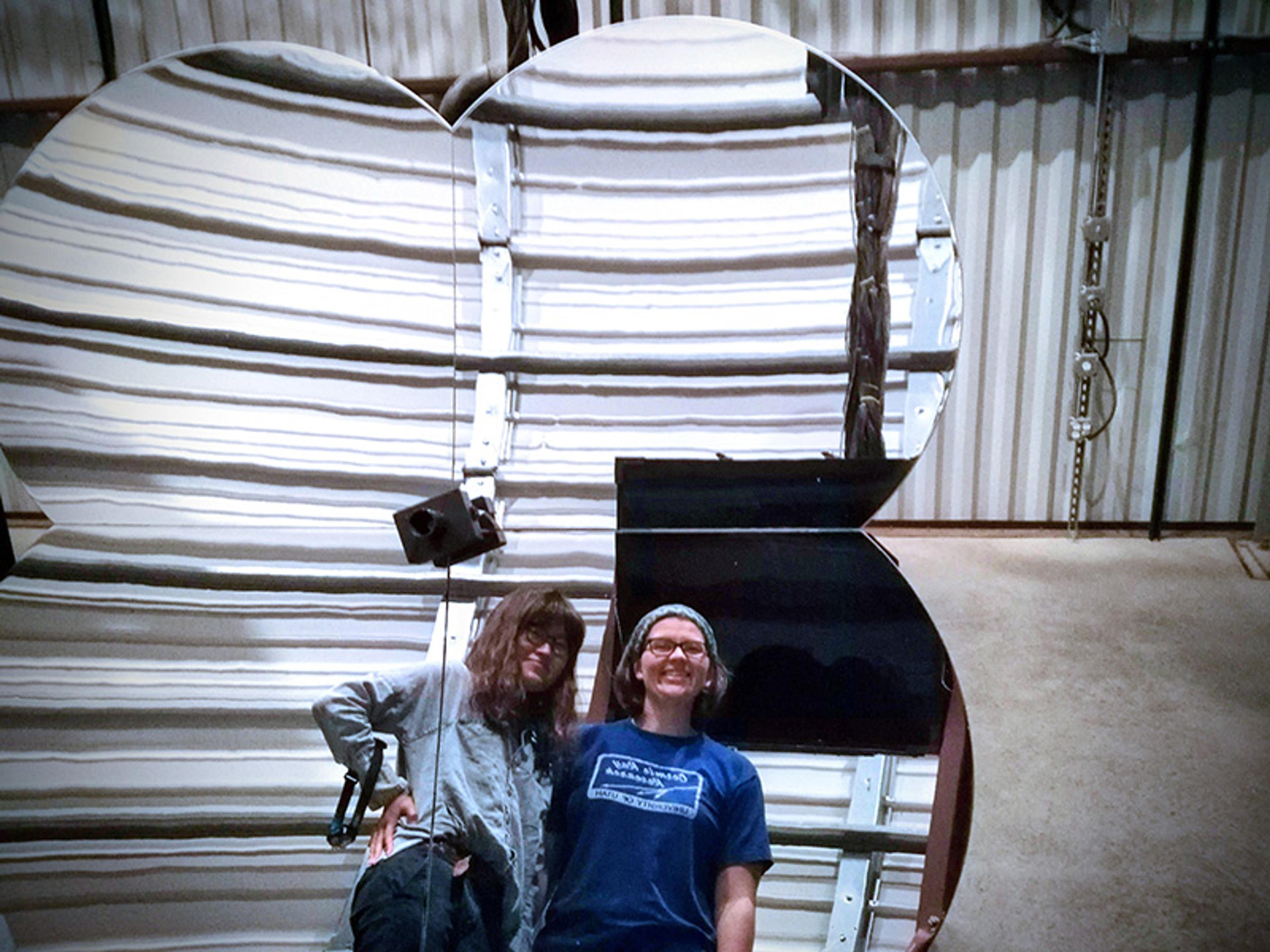
Jihee Kim and Jamie Zvirzdin with a Telescope Array fluorescence detector near Delta, Utah, shed doors safely closed. Photo supplied by the author
Beyond personal anecdotes, the history-based stories we frequently tell in physics model the scientific method itself. Consider the Austrian physicist Victor Hess who, from 1911 to 1912, conducted a series of risky hydrogen balloon flights, the most famous of which reached an altitude of 5,350 metres – about as high as Mount Everest’s Base Camp – to measure radiation intensity in the atmosphere.
As the atmosphere grew thinner and thinner, Hess, with his two-man crew, stared through the eyepieces of two electroscopes. He carefully counted how frequently the electroscope’s hanging fibres lifted, which meant they were detecting radiation from charged particles (ions) in the atmosphere. He found that, at the highest altitude, the atmosphere had 22 to 24 more ions than at ground level, which meant a significant increase in radiation intensity. Hess’s daring – he also did balloon flights at night, to rule out effects from the Sun – led to the discovery of cosmic rays, proving that Earth is constantly bombarded by these high-energy particles from space. For his efforts, he received the Nobel Prize in Physics in 1936.
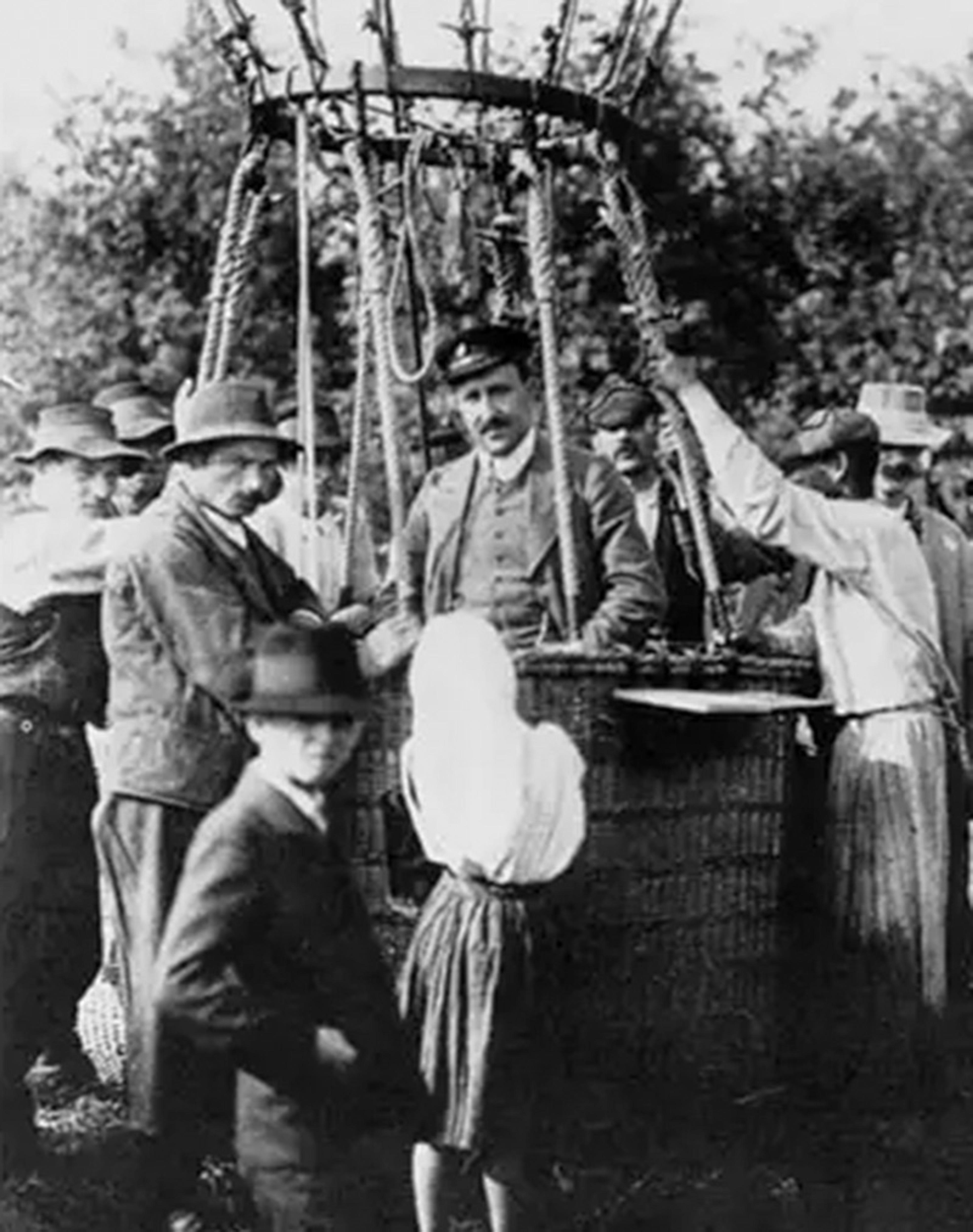
Victor Hess back from his balloon flight on 7 August 1912. Image courtesy of the American Physical Society
This story introduces us to cosmic rays, yes, but also follows the classic progression of a short story: on 7 August 1912, at 6:12am, from Aussig, now the Czech city of Ústí nad Labem (setting), Hess and his crew (characters), curious about this mysterious radiation (exposition) decided to follow a hunch (inciting incident) and go up in a balloon to gather data (rising action, literally), making a groundbreaking discovery (climax), landing safely at 12:15pm in Bad Saarow-Pieskow, Germany, and arriving at important conclusions that were confirmed and rewarded (denouement).
The story’s arc is echoed in the scientific method itself. We start by identifying a problem that needs explanation or further investigation, and we gather research and materials. From a question, we propose a testable hypothesis. We design and conduct an experiment to test the hypothesis, meticulously collecting data and controlling variables as carefully as we can. We analyse and interpret the data to see if it supports or refutes our hypothesis, and from this we draw a conclusion and report our results. It is a satisfying pattern to follow, this roadmap. It is a critical one.
The power of science stories like this lies in their concrete details, an insight that not only helps us be more interesting teachers of physics but also better communicators when reporting our findings. Hess in a balloon, the high altitude, the sensation of flight, the cold metal of the electroscopes – these finite, sensory elements help anchor concepts like cosmic-ray radiation in our minds. As I learned when I did my MFA in writing and literature at Bennington College, using vivid imagery and sensory details – anything you can see, touch, taste, smell, hear – makes new, complex information easier to absorb and remember. As you follow the scientific method, keeping track of these details makes it easier to recount what happened and what you did.
Some stories in physics are straight-up science fiction: aka, thought experiments. These ground abstract concepts in fictional characters, scenarios and sensory details. Take the Twin Paradox, as told by Amber Stuver in an excellent TED-Ed video.
Stuver’s Twin Paradox explanation is the best I’ve yet heard, in contrast to fairly confusing ones out there. Some people don’t know how to tell a good story, perhaps through no fault of their own. It’s worth taking the time to learn how.
The physicist Robert Resnick anthropomorphised Einstein’s story to a travelling twin returning to his brother
As with Hess and his balloon, the Twin Paradox has characters, setting, actions, pacing, concrete details, the works. Once we can properly see the outline of the story, either directly or by imagining it, we can attach formulae like the Lorentz factor (which shows how time slows down for objects moving near the speed of light) and other mathematical details. I now see mathematics equations as sentences in their own right, adding concrete, sensory details that flesh out these stories and even providing fundamental plot points that advance the story.
The characters in thought experiments have taken on lives of their own as the culture of physics has evolved through time: the original Twin Paradox thought experiment came in 1905 from Albert Einstein in the form of some pretty basic clocks. To explain special relativity in his original paper, Einstein wrote about two synchronised clocks, one of which moved from point A to point B along a line. The moving clock story then evolved, as stories do: in 1911, Einstein himself reimagined the travelling clock in terms of ‘a living organism in a box’. The physicist Robert Resnick then anthropomorphised the story to a travelling twin returning to his brother.
A similar evolution happened to my favourite thought experiment, Maxwell’s demon. In 1867, James Clerk Maxwell pictured two compartments linked by an intelligent valve that could sort fast particles from slow particles, but Lord Kelvin embellished the story to include a demon with hands and feet, much to the delight of bored physics students everywhere. The demon selectively allows faster (hotter) molecules to pass one way and slower (cooler) molecules to pass the other, seemingly creating a temperature difference and violating the second law of thermodynamics, which says that the Universe always tends toward chaos. However, the demon’s work requires energy to gather information and sort the molecules. This energy expenditure ensures that the overall entropy of the system still increases, preserving the second law of thermodynamics.
Character archetypes like Alice and Bob make frequent appearances in quantum cryptography, a way of securing communication by applying principles of quantum mechanics to encrypt data. Alice and Bob first appeared in the paper ‘A Method for Obtaining Digital Signatures and Public-key Cryptosystems’ (1978) by the computer scientist Ronald L Rivest et al, where Alice and Bob share a secret encryption key to secure against an eavesdropper (often called Eve). It is fun to have Alice and Bob pop up in different problems. These characters have the additional value of finding their way into popular culture, enticing new people to come to imagine these strange science scenarios.
In his book Knowledge and Error: Sketches on the Psychology of Enquiry (1905), Ernst Mach argued that these imaginary, proxy experiments were ‘a necessary precondition for physical experiment’. Imagining these stories could ‘enliven enquiry, tell us about new properties and promote insight into their connections.’ Such stories – complete with characters, setting, a story arc and sensory details – are tools we use to think through a specific problem deliberately and systematically.
In physics courses, we’re often expected to write formal proofs in our weekly problem sets, showing how one equation evolves into another. We’re shown the beginning and the end of the story, like a flashforward in time, and asked to fill in the plot points that lead to the conclusion, ending as dramatically as ‘The End’ with the initials QED (in Latin, quod erat demonstrandum – what was to be demonstrated).
I really like proofs – I find them satisfying. There is something lovely in the way they sway back and forth between words and equations. A proof, like a narrative, is a carefully crafted sequence of ideas, leading the reader from assumptions and definitions to a logical conclusion. I see physics quantities – Force, Entropy, Volume, Current Density, Energy – as fully fledged characters, each possessing dimensionality (literally: dimensions of mass, length, time, charge – more formally, current – temperature, number of moles, and luminous intensity), affecting how they perform on the page. Their nicknames are their symbols in an equation: F for Force, S for Entropy, V for Volume, J for Current Density, and so on. As in a story with many characters, sometimes it takes a while to learn them all, particularly since everyone has preferred pet names.
But the naming is important. My friend and fellow literary aficionado Pierre Sokolsky, dean emeritus of the University of Utah College of Science, told me: ‘Once you give something a name, you are using language, with everything that implies. The concept “Force”, once stated, has all the power of language to bring up images, similarities, even stories.’ Through the process of naming, physics quantities thus become characters in a grand story of not just the proof but the Universe. Each quantity shapes and is shaped by the natural laws it obeys.
In a successful story, all main characters must evolve in some way; they must be subjected to processes that reveal their fundamental nature as the proof unfolds. The same is true with physics quantities. Since more than one quantity is usually involved, the relationships between multiple characters deepen and become more complex; their combinations and machinations produce new versions of themselves.
Ultimately, both story and proof lead the reader on a journey towards understanding
Some textbook writers and teachers drive me bananas when they treat their physics characters on the page like a shell in the shell game: they shuffle quantities around as fast as possible, switching characters and perspectives without care. Certain physics quantities are renamed willy-nilly with the most squiggly Greek characters possible, which is as jarring as renaming the main character in your story without bothering to signal the change to the reader or explain why. Instead of illuminating the deeper connections between physical quantities, poor physics communicators obscure rather than explain what they’re doing, robbing the process of the intellectual and narrative clarity that makes physics so compelling. If you don’t explain what is happening, even briefly, or if you skip too far forward in your proof, your reader will quickly grow frustrated and lose interest in your narrative. If your reader is your teacher, you’ll lose points. If your reader is a grant-giver, you won’t get your grant.
The warp and weft of proofs, weaving words with numbers, sentences with equations, became familiar to me while editing formal proofs for Taylor & Francis. It was my job to ensure that the equations were properly punctuated when part of a sentence. Beyond keeping track of your characters, you need clarity, precision, structure and progression. These are all skills learned when studying language arts. Ultimately, both story and proof lead the reader on a journey towards understanding, closure and insight about the Universe.
Physicists love metaphor, even if they claim otherwise. The Italian rhetorician Giambattista Vico in the 1700s called the metaphor ‘a fable in brief’. Metaphors and their relatives – comparisons, similes, analogies – are far more important in physics than you might think. An astute metaphor – a mini-story – can be the beginning of understanding a concept in physics, even the beginning of a new field of enquiry, as Michael Faraday’s analogies ‘current’ and ‘lines of force’ did for electromagnetics.
Beyond sheer repetition of stories and cultural exposure to mathematics and physics concepts – which not everyone has the privilege of receiving, particularly if you had a heavy-handed Third Culture – metaphors and similies are the primary way humans learn. We connect something we do know to something we don’t. Here’s an example: a Fourier analysis is like turning a symphony into its individual musical notes. Just as we could break down a complex orchestral performance into distinct instruments, Fourier analysis allows us to decompose a complicated signal or waveform into simpler wave shapes. Here’s another example: working with physicists whose egos are bigger than supermassive black holes is like poking yourself in the eye with a needle, over and over.
I am guilty of loving metaphors. I’m not sorry. To me, they breathe life, light and colour back to that which was deadly boring. When I become bored, I stop paying attention, so I try to fight this inclination by at least amusing myself with metaphors. In my book Subatomic Writing (2023), where I compare two traditionally dry subjects (grammar and particle physics), I liken particles of language to particles of matter and, through six lessons, build from word to paragraph as we build from a quark to a chain of carbon atoms – a pencil’s graphite. The ability to create such mini-stories as I learn is, for me, part of why I’ve been able to level up quickly in physics.
In cosmic ray science, metaphors play a key role, too. For example, the Oh-My-God particle had an energy of 3.2 × 1020 electronvolts. To explain this quantity to those unfamiliar with units of energy like electronvolts and Joules, we used analogies: the OMG particle had the same kinetic energy as a bowling ball dropped from shoulder height, or a baseball thrown at 28 metres per second (63 miles per hour). We also describe ultrahigh-energy cosmic rays as ‘tiny bullets’ striking Earth’s atmosphere with incredible force. These analogies not only simplify complex phenomena but also help convey the scale and impact of these particles in a way that resonates with both scientists and the public.
By connecting the unfamiliar with the familiar, metaphors make it easier to internalise and recall new information
These kinds of analogies light up our brains. According to the article ‘The Neural Career of Sensory-motor Metaphors’ (2011) by the cognitive neuroscientist Rutvik H Desai et al, metaphors engage the neural networks of the brain that deal with sensory processing, motor planning, abstract thinking, emotion and memory. They do excellent things to keep us awake and engaged, these mini-stories.
In other words, metaphors bridge abstract concepts and sensory experiences, allowing our brains to process complex ideas more naturally. By connecting the unfamiliar with the familiar, metaphors make it easier to internalise and recall new information, which is why they are such powerful tools in teaching and learning, especially in subjects like physics.
But metaphors can also skew our thinking about a phenomenon. Beau Biller is a forensic mechanical engineer and an assistant instructor for the Johns Hopkins applied physics programme. He sees many students wrestle with difficult physics concepts. In the early stages of studying Einstein’s theory of general relativity, teachers often help students ‘see’ the curvature of space by showing them a rubber sheet with a bowling ball in the middle. Biller told me:
It is very difficult to make analogies for the geometry in which we live. As far as we know, we’re not on a four-dimensional rubber sheet embedded in a higher dimension that we can ‘look in’ upon … Much like learning a new language, some of the concepts encountered in modern physics are simply … hard. No shortcuts allowed.
All the same, metaphors can approximate a difficult concept. They are rough models that can be modified the more we learn. Maxwell, one of my favourite physicists, used billiard balls as a starting point to explain the interaction of molecules in his book Theory of Heat (1871), but he also explained that ‘two hard spherical balls is not an accurate representation’. He went on to explain why and relabelled this interaction as an ‘Encounter’, modifying the mental metaphor.
At the beginning of each semester of my Master’s in applied physics, I think of a reigning metaphor I can use to learn the upcoming subject matter. During quantum mechanics last semester, since I often play the computer game ARK: Survival Evolved with my brother on Sunday afternoons, I started with the axiom ‘A particle is like a triceratops named Alice.’ As silly as it sounds, it was enjoyable and memorable. It was a fable that gave me a rough outline of the story of quantum particles and their interactions. I studied hard, wrote down all my dinosaur-related metaphors in detail, particularly mathematics details, in an Overleaf document just for my own sake, and experienced the pleasurable shock of earning an A+ in quantum mechanics.
Fun and helpful mnemonic aids aside, physics is most thrilling when we can pull away the scaffolding of metaphors and see mathematics itself as the storytelling framework. ‘We have to read the story behind the equation,’ as Biller said, and I fully agree. The deeper we go in physics, the more the language of mathematics empowers us to precisely narrate the epic tale of the Universe.
Some stories in physics are downright Kafkaesque – like the Monkey and Hunter thought experiment, which teaches projectile motion at great cost to the monkey; or Schrödinger’s Cat, which is forever being murdered or not murdered in a box containing a vial of poison.
Just as Schrödinger’s Cat demonstrates quantum paradoxes, the man behind this thought experiment embodies the uncomfortable paradox of a brilliant mind who nevertheless chose to engage in predatory behaviour towards young girls, acts documented in his own diary. Erwin Schrödinger groomed a 14-year-old girl he was tutoring and impregnated her when she was 17. The abortion made her sterile.
Richard Feynman’s FBI files, released in 2012, show the physicist’s private behaviour did not match his playful, charming public persona. One page of the report says:
His ex-wife reportedly testified that on several occasions when she unwittingly disturbed either his calculus or his drums he flew into a violent rage, during which time he choked her, threw pieces of bric-a-brac about and smashed the furniture.
Feynman also told boastful stories of frequenting strip clubs and having manipulative approaches to women in his earlier years.
Hero worship in physics culture becomes insidious when we refuse to challenge unethical behaviour
In his diary, Einstein recorded wildly racist things about people from China and other countries when he visited the Far East and the Middle East in 1922-23. He cheated on his first wife. And his second wife. The list of bad behaviour by intellectual elitists goes on. This grim reality reminds us that the emotional and physical abuse perpetrated by physics ‘geniuses’ has been catastrophically downplayed – a trend we must confront and reform.
The problem of hero worship in physics culture becomes especially insidious when we refuse to challenge unethical behaviour in revered figures, allowing misconduct to persist unchecked. It is crucial to confront these darker narratives, not to diminish the scientific contributions of these individuals but to ensure that harmful legacies don’t continue to thrive in our institutions.
Other sombre stories serve to promote empathy rather than tyranny. Sokolsky told me his favourite physics tool is a hammer, ‘to remind me’, he says, ‘to allow students to finish their PhD theses.’ He alludes to the 1978 incident where a Stanford mathematics student, Theodore Streleski, killed his faculty advisor with a hammer after failing to complete his dissertation for 19 years.
The narratives we construct about our abilities and challenges in life are as vital as the equations we solve. ‘Being a stubborn/persistent scholar who loved the process of understanding how everything works has been key,’ says Rasha Abbasi, an astroparticle physicist at Loyola University Chicago. I’ve known Abbasi since she was a PhD student – and I, a 16-year-old intern – studying cosmic rays at the University of Utah. Today, she studies gamma-ray flashes from lightning with our cosmic ray detectors in the Utah desert. We’ve kept in contact through the years, and she inspires me with her tenacity, good nature, humour and intellect. When I ask Abbasi if she has any thoughts on the role of language in physics, she says: ‘I discovered later on in my career that language is a big part of being a scientist. Training in writing and communication needs to be emphasised more in our field.’
She’s right. Physics students can forget writing is a critical part of being a physicist: there are white papers, reports, journal articles, National Science Foundation grants, posters, presentations. Every type of writing involves some connection to story, even if the character of your story is a variable in an equation.
In the end, the stories we tell shape our trajectories in life as profoundly as the cultural forces that mould us, serving as both barriers and bridges to our greatest ambitions. I have found a healthy balance among the cultures I subscribe to, and ambition is no longer a dirty word. I want to work with my friends to uncover the origins of ultrahigh-energy cosmic rays, a longstanding mystery. I want to change the way we teach physics. I want to win a Nobel Prize. My story of finding physics again is over, but the story of what I’ll do with it has just begun, and I’m excited to see what happens next.
In political science, supporters of ‘horseshoe theory’ believe that far-Left views and far-Right views are more similar to each other than they are to more moderate, centrist views. Perhaps there exists a corollary between literary writing and physics, an academic horseshoe theory. You will find me happily oscillating back and forth in the cheerful space between Dictionopolis and Digitopolis, building bridges and repairing fences. I invite you to step out of your comfort zone, continue to confront and conquer challenging material, and join me in building the City of Wisdom, one story at a time.
This Essay was made possible through the support of a grant to Aeon Media from the John Templeton Foundation. The opinions expressed in this publication are those of the author and do not necessarily reflect the views of the Foundation. Funders to Aeon Media are not involved in editorial decision-making.